The dangers of blue carbon offsets: from hot air to hot water?
Authors
Share
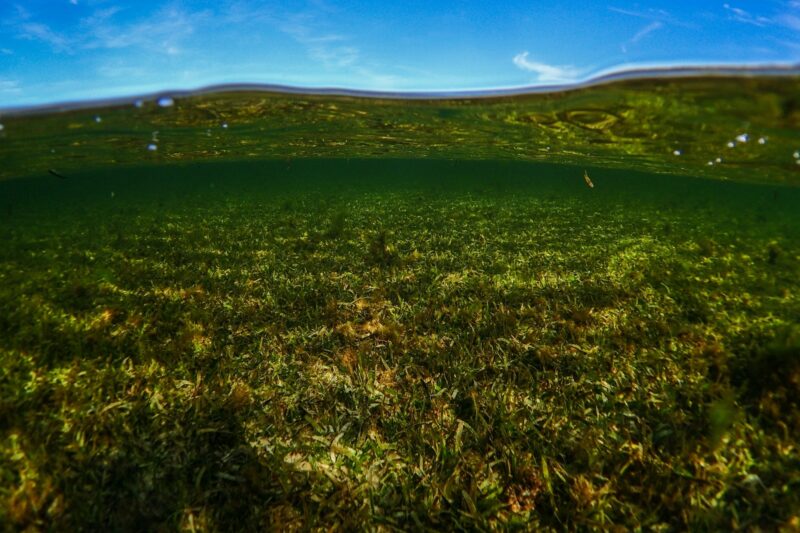
There has been much talk of “Blue Carbon” at the Bonn climate negotiations COP23. But what does it really mean? This briefing sets out the issues and finds that the use of blue carbon to offset and hence effectively avoid required emission reductions in other sectors would undermine our ability to limit temperature rise to 1.5°C.
Issue
The use of blue carbon to offset and hence effectively avoid required emission reductions in other sectors such as fossil fuel combustion, industry, agriculture, international aviation and marine activities would undermine our ability to limit temperature rise to 1.5°C. There is very little room for offsetting in mitigation pathways compatible with the Paris Agreement due to the very limited carbon budget, yet there are already attempts to use the land sector and now the coastal ecosystems to contribute to national mitigation targets and reduce the emissions reductions needed in other sectors. This is particularly concerning for coastal ecosystems as carbon measurements are highly uncertain and carbon sequestration may, or is even likely to, be reversed by the increasing impacts of climate change.
Key points
- Blue Carbon is a term that refers to the carbon sequestered in coastal ecosystems – namely mangroves, sea grasses and salt marshes. Large areas of these ecosystems have already been deforested or degraded by human activities leading to carbon emissions.
- The conservation and restoration of coastal ecosystems is essential for the preservation of their essential ecosystem services, which range from supporting fisheries to providing coastal protection to carbon sequestration. In addition, healthy ecosystems tend to be much more resilient to the impacts of climate change.
- The challenge with Blue Carbon is that the term focuses on the climate sequestration component of coastal ecosystems within a mitigation context. This is problematic for a number of reasons: Carbon flows within the highly variable environmental in the coastal zone are very difficult to measure, particularly in developing countries, so estimates of carbon sequestration are highly uncertain. In addition, it is very difficult to determine which emissions and removals are natural and which are anthropogenic.
- The impacts of climate change and other stressors (both human and natural) can damage coastal ecosystems and may reduce their resilience in the long-term, leading to a reversal of carbon sequestration.
- flows using Blue Carbon to achieve national mitigation targets risks diluting mitigation ambition in other sectors. Lessons from the land-use and forestry sector show how countries have already used imaginative accounting schemes to allow countries to offset their fossil fuel emissions. There is very little room for such offsetting in mitigation pathways compatible with 1.5°C, especially since Blue Carbon offsets come with high uncertainties and risks of reversal, and because their additionality (i.e. whether emissions reductions or removals would have happened without the Blue Carbon project) can be difficult to prove.
- Any attempts to measure and set targets for carbon sequestration in coastal ecosystems should be kept separate from emissions targets in other sectors. Lessons from LULUCF have shown that integrating nature-based mitigation offsets under national mitigation targets creates loopholes, hot air, and MRV challenges. A more verifiable option would be to set targets for, for example, reducing deforestation and degradation rates, or protecting or restoring a certain area of coastal land.
- It is essential to prevent the degradation of coastal ecosystems, but carbon sequestration is not necessarily the most valuable ecosystem service for local populations. The wealth of potential co-benefits from coastal ecosystem conservation and restoration, beyond carbon sequestration, are justification enough for schemes to incentivise their protection (e.g. Payment for Ecosystem Services, or PES) in developing countries. If well managed, such schemes could have substantial adaptation benefits for local communities, in addition to other ecosystem services. To maximise these benefits, schemes should be designed with the full set of ecosystem services in mind, not just carbon sequestration.
What is Blue Carbon?
Blue Carbon refers to the carbon sequestered in coastal ecosystems – namely mangroves, sea grasses and salt marshes. Estimates of the total amount of carbon stored globally in these natural ecosystems globally are highly uncertain, as are estimates of the carbon sequestered each year through natural processes.
Mangroves are being deforested at an alarming rate, although there is also uncertainty in the extent of deforestation (0.2% – 2%) (Atwood et al. 2017; Alongi & Mukhopadhyay 2015) and in the associated emissions. Indonesia accounts for about half of mangrove deforestation, the next most significant countries being Malaysia, the U.S. and Brazil (Atwood et al. 2017). Eliminating mangrove deforestation in Indonesia and Malaysia alone could reduce global soil carbon emissions from mangrove deforestation by ~70%. The IPCC’s AR5 report gives a range for the estimated carbon loss of 70-420 MtCO2/yr; at its upper end, this could represent as much as 10% of emissions from deforestation (Donato et al. 2011).
The coastal ecosystems associated with Blue Carbon provide numerous other crucial services, both for people and for biodiversity. Increasing attention is being paid to the need to conserve and protect these vital ecosystems, and this has led to discussions over the use of Blue Carbon to incentivise such efforts. However, a focus on the carbon sequestered in coastal areas risks distracting from the need to urgently mitigate emissions in other sectors, as well as the need to preserve the life-supporting ecosystem services that coastal ecosystems provide.
Oceans are high on the agenda this year. A UN Oceans Conference was held in New York in June, and the UNFCCC COP23 Presidency has launched its proposal for an Ocean Pathway as an initiative to bring ocean issues into the international climate change process. A number of countries have proposed including Blue Carbon into NDCs for mitigation offset purposes. This means it is essential that the key challenges and risks associated with the Blue Carbon concept are well understood.
What are the risks of using Blue Carbon to offset emissions?
There are three fundamental problems of using mitigation through land or ocean-based natural systems to offset CO2 emissions: uncertainties, climate change impacts, and the risk of offsetting. These are described below.
Uncertainties
The uncertainties in the measurement of carbon flows in coastal systems are much higher than the uncertainties in emissions in other sectors. There are also uncertainties over the permanence of ocean-based mitigation, and the risk of leakage of emissions through coastal change elsewhere. These uncertainties, described in greater detail below, mean that mitigation using coastal ecosystems cannot be treated like-for-like with other forms of mitigation.
1. Changes over time
Tropical mangrove forests change over time. Mangroves are non-linear systems that are not in equilibrium, as they have adapted to grow on continually evolving shorelines. The amount of carbon sequestered by mangroves and other coastal ecosystems varies substantially between locations and as a function of time. While mangroves are often described as being far more intense carbon sinks than tropical forests, some mangrove systems may not actually accumulate any carbon. For example, a survey of 17 Australian seagrass habitats revealed an 18-fold difference in the carbon stored (Lavery et al. 2013).
2. Measurement uncertainties
The measurement of carbon storage is difficult and should be interpreted cautiously. Adame et al. (2017) compared estimates of mangrove root biomass calculated using allometric equations with values measured in the field and found field measurements to be on average 40% smaller (with some samples showing a difference of over 1000%). Another challenge is that a number of processes in coastal systems are poorly understood. For example, the fate of carbon once it has been deposited in a coastal area is still unknown (Atwood et al. 2017).
3. CO2 effects
Mangroves and seagrasses may experience enhanced productivity due to higher CO2 levels (IPCC AR5). Distinguishing this effect from human interventions to enhance productivity and thus carbon storage will be difficult.
4. Permanence and leakage
As with other land-based sequestration, ensuring permanence and preventing leakage is very difficult. Conservation of one area may displace deforestation or degradation to another area, leading to leakage, and ensuring the longevity of carbon stored in conserved or restored mangroves requires a strong MRV system (Murray et al. 2009).
Impacts
The impacts of climate change on coastal ecosystems may lead to a reversal of carbon sequestration in the future. Mangroves and sea grasses are vulnerable to the impacts of climate change, such as ocean warming, sea level rise and increased storminess (Ward et al. 2016), as well as to other human drivers. These impacts are expected to worsen in the future as more greenhouse gases are emitted.
However, in some regions coastal ecosystems may benefit from climate change, at least in the short-term. For example, higher precipitation could lead to greater sediment and nutrient input in some Pacific Islands. Such impacts – both positive and negative – could affect carbon storage (IPCC AR5 (2013)). Emissions may go unaccounted, or removals may be overestimated or reversed. The regional variation in climate impacts will make the design of any system for accounting for natural disturbances very difficult.
1. Sea level rise
Research has shown that coastal ecosystems in some regions are vulnerable to sea level rise. If sediment supply is sufficient for mangroves to grow in pace with sea level then ecosystems can continue to survive and to sequester carbon, but in many regions sediment supply is not sufficient (Lovelock et al. 2015). For example, in the Indo-Pacific – home to over half of the world’s mangrove forests – sediment delivery is declining, largely due to human activity, e.g. damming rivers. Mangrove forests at sites with low sediment supply and low tidal range – such as the Gulf of Thailand and the Solomon Islands – are particularly vulnerable, and could be submerged by 2070 under even a low level of sea level rise (Lovelock et al. 2015).
2. Sea level fluctuation
In some regions sea level can fluctuate with changes in rainfall. For example, the Indo-Pacific experiences low rainfall and low sea levels during El Niño, and this can lead to salinization of the soil and mangrove mortality (Lovelock et al. 2017). Intensification of ENSO under climate change and associated fluctuations in sea level may lead to mangrove degradation and carbon release, and may further increase mangrove vulnerability to other impacts of climate change, pollution, and other human influences (Lovelock et al. 2017).
3. Ocean warming
Ocean heat waves have already had severe impacts on coastal ecosystems. For example, for a four-month period in 2010/2011 sea temperatures in western Australia were 2-4°C above average, causing 90% die-back of sea grass beds in the Shark Bay World Heritage Area in some areas. Two years later, these ecosystems had only partially recovered (Nowicki et al. 2017). More recently in 2016, the extreme El Niño event led to a very extensive dieback of mangroves in the Gulf of Carpentaria in northern Australia (Duke et al. 2017). Such warming events are projected to become more common in the future, which is particularly concerning given the slow recovery rates of many coastal ecosystems.
4. Interacting stressors
Many coastal species are able to cope with highly variable environments, but the interactions of multiple stressors can amplify negative impacts and drive ecosystems into alternative states. For example, one study found that a marine heatwave in combination with a flooding on land leading to sediment discharge and high water turbidity in the near shore marine environment in western Australia resulted in loss of seagrass biomass both
above and below ground, and ultimately reduced the resilience of the ecosystem to future disturbance (Fraser et al. 2014).
5. Potential benefits for carbon sequestration
Increased CO2, warmer temperatures and moderate increases in sea level can increase rates of plant productivity and carbon sequestration. However, these same drivers can also act to reduce the carbon pool, for example, warmer temperatures and increased CO2 can also increase decay rates, lowering the rate of carbon sequestration. How these factors will play out in the future, especially in the context of the negative impacts outlined above, is very uncertain.
Offsetting
Offsetting reduces the ambition of mitigation in other sectors. Previously insufficient mitigation action means that a substantial volume of negative emissions will be needed in addition to ambitious action in all other sectors, so there is no space in emissions pathways for the use of mitigation from nature-based systems to offset emissions in other sectors, unless those emissions are prohibitively expensive to mitigate.
Use of Blue Carbon as an offset against the necessary reduction of CO2 and other GHG emissions from fossil fuels, industry and agriculture will not help achieve the global emissions pathway need to meet the Paris Agreement‘s 1.5°C warming limit.
The use of Blue Carbon to offset emissions is particularly concerning because of its uncertainties. Any movement of carbon credits and debits between nature-based systems and other sectors risks leaking these high uncertainties to mitigation in other sectors, making it difficult to monitor progress and to ensure that action is in line with the Paris Agreement.
Lessons learned from LULUCF accounting
A number of relevant lessons have been learned from the land-use, land-use change and forestry (LULUCF) sector:
- The development of accounting rules for the LULUCF sector under the Kyoto Protocol allowed Parties to develop complicated rules, which are beyond the understanding of most policy makers, scientists and members of civil society. These rules have enabled some emissions to go uncounted towards national emissions targets, creating hot air that undermines the need for rapid emissions reductions.
- For example,under Article 3.7 any country that had land-use emissions in the base year (1990) can include these emissions in their reference year emissions when calculating their reduction target. Any decreases in emissions excluding land-use relative to the base year including land-use emissions are awarded. In Australia for example, a substantial decrease in deforestation rates since 1990 is allowing Australia to comply with its 2020 Kyoto target when accounting rules are applied, despite a continued increase in fossil emissions (Climate Action Tracker 2015, 2017).
- Now countries vary widely in how they incorporate the LULUCF sector in their NDCs, which leads to considerable uncertainty in emissions reduction targets (Grassi & Dentener 2015), particularly where Parties have chosen to include LULUCF in their overall emissions target (i.e. gross-net accounting) or plan to use accounting against an unspecified reference level. The addition of Blue Carbon into the NDCs would further increase this uncertainty.
- The EU is currently negotiating its accounting rules for LULUCF under the EU’s climate and energy package. A number of loop-holes have been proposed to enable countries to use LULUCF credits to offset action in other sectors, and to allow increased levels of wood harvesting to continue without the associated emissions being accounted (CAT 2017). These loopholes, if approved, could reduce the ambition of the EU’s climate targets.
- What are the alternatives?
Coastal ecosystems are threatened by a number of stressors: coastal infrastructure, tourism, agriculture, aquaculture, dam development, pollution, and overfishing, among others. Over the past quarter-century mangrove forests have declined by more than 30% (Duke et al. 2007), illustrating the severity of the problem.
This rapid rate of degradation is particularly concerning because mangroves and other coastal ecosystems are vital for coastal communities, providing essential food and resources as well as coastal protection and erosion reduction. It is these benefits of coastal ecosystem restoration that should drive conservation and restoration efforts.
It is difficult to generate economic incentives for conservation of coastal ecosystems because many of the ecosystem services that they provide do not have a market price, and often decisions that result in their degradation are made by bodies for whom the value of mangroves is non-existent (Mukherjee et al. 2014). To provide an incentive for the preservation and sustainable use of these ecosystems, Payment for Ecosystem Services (PES) schemes can be used to place a value on those services that are valuable to local communities.
The wealth of services provided by mangroves and other coastal ecosystem makes them well-suited for such schemes. One estimate for the annual economic value of mangrove loss from aquaculture came to US$4-17 billion (Mukherjee et al. 2014), and this likely underestimated the value to local populations of a number of benefits. Notably, the same study showed that carbon sequestration does not rank highly in terms of economic value or expert-based valuation. Numerous other ecosystem services are more important for local communities: food, livelihoods, construction materials and coastal protection provide essential life support services, and it is these services that should be driving the protection of coastal ecosystems.