What’s on the science agenda for the IPCC Special Report on 1.5°C in agriculture
Delphine Deryng
Since the adoption of the Paris Agreement with its 1.5°C temperature limit, climate scientists are preparing a comprehensive assessment of the impacts of climate change under 1.5ºC warming in global average temperature. Here’s an overview of what we already know and what we would need to learn from the IPCC Special Report on 1.5°C due in 2018 regarding impacts on agriculture.
Share
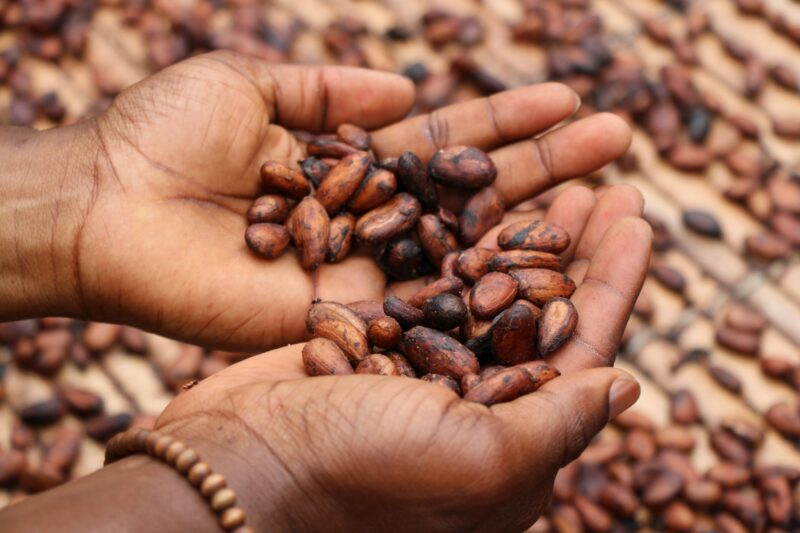
Climate science, and importantly IPCC Assessments, have underpinned the UNFCCC climate negotiation process from the outset. Over the last 30 years, scientists have researched climate impacts, adaptation and vulnerability but focused largely on quantifying impacts under much hotter high CO2 climates, which would result from a business as usual emissions scenario, and reporting future impacts for time periods such as the 2050s or 2080s. Until recently, researchers have largely overlooked impacts under much lower levels of global temperature and CO2 rise – such as 1.5ºC.
Adoption of the 1.5°C temperature limit in the Paris Agreement has surprised many in the scientific community. But now climate scientists are preparing a comprehensive assessment of climate change impacts under 1.5ºC above pre-industrial conditions. There’s already a body of research on the differences in climate impacts on agriculture between 1.5°C and 2°C warming and how climate change and variability affects crop production.
Following the approach used in the food chapter of IPCC Fifth Assessment Report, all pillars of food security – chiefly food production, access, stability and utilisation – must be addressed to provide a comprehensive assessment of the consequences of the 1.5ºC target for food security at global and regional levels. For instance, along with declines in the supply of staple food, production losses for cash crops can severely impact poverty and access to sufficient and nutritious food in many parts of the world.
Other assessments, such as the World Bank’s Turn Down The Heat series of reports showed that often regions that are already highly vulnerable to climate variability and poor, such as the Least Developed Countries (LDCs) and many Small Island Developing States (SIDS) are at risk: “…global warming above 1.5°C to 2°C increases the risk of reduced crop yields and production losses in Sub-Saharan Africa, South East Asia and South Asia. These impacts would have strong repercussions on food security and are likely to negatively in negatively influence economic growth and poverty reduction in the impacted regions.”
What we know
Agricultural production is particularly sensitive to climatic conditions and limiting the increase in global average temperatures to 1.5ºC above pre-industrial levels in the coming decades will certainly avoid large production losses that would otherwise occur from higher increases around the world. Recent climate impacts literature already shows that the overall negative impacts under 1.5ºC are much less pronounced than under 2ºC warming above pre-industrial levels, especially for key cereal crops such as maize and wheat grown in the tropics (Schleussner et al 2016). Stabilising global average temperature increase to 1.5ºC could halve the risk of extreme heat stress events, which can have a large negative effect on crop production, in sub-Saharan Africa (Serdeczny et al 2016).
Projecting the effects of climate change on crop production entails large uncertainties. The range of uncertainty in climate impacts projections for any given climate change scenario is substantial and this combines almost multiplicatively with the range of uncertainty resulting from future climate change projections under different emission pathways. As a consequence, it has often been assumed in the past that the difference in impact between 1.5°C and 2°C warming would be very small or, in a sense, not detectable.
More recent work comparing results under 1.5ºC and 2ºC found the range of uncertainty in crop modelling projections is reduced by nearly a third, and that there is a significant and statistically detectable difference in impact between these two levels of warming (Schleussner et al 2016).
Recently developed high-resolution global agricultural datasets have enabled scientists to analyse observed crop-climate variability relationships. 20-40% of yield inter-annual variability over the last 40 years can be attributed to climate variability, depending on crop types (Ray et al 2015, Iizumi and Ramankutty 2016), and there is regional evidence that the significance of this relationship has increased over the last 20 years (Trnka et al 2016).
Droughts and extreme heat stress events in particular – but less so floods and extreme low temperatures – have been responsible for 9-10% losses in national crop production over the last 40 years (Lesk et al 2016).
More recent drought events have doubled the negative impacts on crop production. Relative losses appear to be more substantial in developed agricultural systems facing up to 20% production deficit directly attributed to droughts, compared to 12% and 9% in Asia and Africa, respectively.
Research gaps
Here’s what we would still need to learn from the IPCC Special Report on 1.5°C regarding the agricultural sector:
- Effects of extreme weather events on future crop production
- Climate impacts on cash crops like cotton, coffee and cocoa
- How climatic conditions influence pests and diseases proliferation and pollinator extinction
- The role of elevated atmospheric CO2 concentrations and ozone on crop production
- Role of CO2 on weeds and competition between crops and weeds
- Farmers’ adaptive capacity
Only a few studies have reported on the effects of extreme weather events on future crop production. This was a major gap in earlier IPCC assessments due to a lack of scientific literature. The new literature developed, as well as work underway in the scientific community (which needs to be accepted for publication by October 2017 to be included in the 1.5°C Special Report) will hopefully ensure that that the 2018 IPCC 1.5 Special Report can at last address this issue.
The risk of extreme temperatures is projected to increase with rising mean temperatures and will impact both large crop producing areas, such as the US Midwest and the Chinese wheat belt, and lower income countries located in sub-tropical and tropical areas (Deryng et al 2014). Mitigation efforts to drastically reduce greenhouse gas emissions to stabilise global temperature below 2ºC could avoid more than 80% of crop losses otherwise projected under a business as usual emission scenario (Deryng et al 2014, Müller et al 2015).
New studies focusing on the benefits of holding global temperature increase to 1.5ºC will likely show further benefits compared to 2ºC, with respect to the role of extremes. In fact, global wheat production is estimated to fall by 6% for each degree of further temperature increase (Asseng et al 2015), with much larger decreases in many of the most vulnerable regions.
Researchers have also largely overlooked climate impacts on cash crops, such as cotton, coffee and cocoa, despite the importance of these crops for livelihoods and thus development and food security in the tropics and sub-tropics (Bunn et al 2014).
Along with direct impacts of changes in mean climatic conditions and extreme weather events, indirect effects on agricultural production related to pests and diseases proliferation (Bradshaw et al 2016) and pollinator extinction (Potts et al 2016, Kerr et al 2015, Settele et al 2016) present another gap in the literature.
The role of rising elevated atmospheric carbon dioxide concentrations (CO2) and ozone (O3) exposure on crop yield remain another source of uncertainty. In the case of CO2, recent studies point to lower beneficial effects for crops grown where water is limited (Gray et al 2016) and expected decrease in the nutritious quality of cereal crops (Myers et al 2014). O3 is a pollutant gas detrimental to crop yield and is a serious threat, especially for Chinese and Indian agriculture, where current O3 emissions are high (Ainsworth 2016).
Looking at adaptation of agricultural systems from a biophysical angle, (Korres et al 2016) recently highlighted the role of CO2 on weeds and competition between crops and weeds. Plants have different ways of producing biomass, a process that requires light, CO2 and water. Typically, plants follow either the C3 or C4 photosynthetic pathway, the latter being more water use efficient but less responsive to CO2. Different CO2 effects on C3 vs C4 plant types will bring different challenges for adapting to a CO2-rich atmosphere across regions. For instance, C3 crops (such as wheat and rice) will compete better against C4 weeds due to the greater CO2 effects on photosynthesis rates of C3 crops – but similarly C4 crops (such as maize and sorghum) will face greater threats from C3 weeds. Achieving mitigation to 1.5ºC could however reduce the risks posed by and uncertainty related to the CO2 fertilisation effects.
Last but not least, following recent findings on climate change adaptation in sub-Saharan African agriculture (Rippke et al 2016, Islam et al 2016) for instance, new research needs to better address farmers’ adaptive capacity and assess the challenges posed by different warming and associated impacts. By engaging closely with stakeholders to design meaningful portfolios of options at the regional scale, this research would be a meaningful contribution to formulating climate adaptation policy (Campbell et al 2016).
References
Ainsworth E A 2016 Understanding and improving global crop response to ozone pollution The Plant Journal
Asseng S, Ewert F, Martre P, Rötter R P, Lobell D B, Cammarano D, Kimball B A, Ottman M J, Wall G W, White J W, Reynolds M P, Alderman P D, Prasad P V V, Aggarwal P K, Anothai J, Basso B, Biernath C, Challinor A J, De Sanctis G, Doltra J, Fereres E, Garcia-Vila M, Gayler S, Hoogenboom G, Hunt L A, Izaurralde R C, Jabloun M, Jones C D, Kersebaum K C, Koehler A K, Müller C, Kumar S N, Nendel C, O’Leary G, Olesen J E, Palosuo T, Priesack E, Rezaei E E, Ruane A C, Semenov M A, Shcherbak I, Stöckle C, Stratonovitch P, Streck T, Supit I, Tao F, Thorburn P J, Waha K, Wang E, Wallach D, Wolf J, Zhao Z and Zhu Y 2015 Rising temperatures reduce global wheat production Nature Climate Change 5 143–7
Bradshaw C J A, Leroy B, Bellard C, Roiz D, Albert C, Fournier A, Barbet-Massin M, Salles J-M, Simard F and Courchamp F 2016 Massive yet grossly underestimated global costs of invasive insects Nature Communications 7 12986
Bunn C, Läderach P, Rivera O O and Kirschke D 2014 A bitter cup: climate change profile of global production of Arabica and Robusta coffee Climatic Change 129 89–101
Campbell B M, Vermeulen S J, Aggarwal P K, Corner-Dolloff C, Girvetz E, Loboguerrero A M, Ramirez-Villegas J, Rosenstock T, Sebastian L, Thornton P and Wollenberg E 2016 Reducing risks to food security from climate change Global Food Security
Deryng D, Conway D, Ramankutty N, Price J and Warren R 2014 Global crop yield response to extreme heat stress under multiple climate change futures ERL 9 034011
Gray S B, Dermody O, Klein S P, Locke A M, McGrath J M, Paul R E, Rosenthal D M, Ruiz-Vera U M, Siebers M H, Strellner R, Ainsworth E A, Bernacchi C J, Long S P, Ort D R and Leakey A D B 2016 Intensifying drought eliminates the expected benefits of elevated carbon dioxide for soybean Nature Plants 2 16132
Iizumi T and Ramankutty N 2016 Changes in yield variability of major crops for 1981–2010 explained by climate change ERL 11 034003
Islam S, Cenacchi N, Sulser T B, Gbegbelegbe S, Hareau G, Kleinwechter U, Mason d’Croz D, Nedumaran S, Robertson R, Robinson S and Wiebe K 2016 Structural approaches to modeling the impact of climate change and adaptation technologies on crop yields and food security Global Food Security 10 63–70
Kerr J T, Pindar A, Galpern P, Packer L, Potts S G, Roberts S M, Rasmont P, Schweiger O, Colla S R, Richardson L L, Wagner D L, Gall L F, Sikes D S and Pantoja A 2015 Climate change impacts on bumblebees converge across continents Science 349 177–80
Korres N E, Norsworthy J K, Tehranchian P, Gitsopoulos T K, Loka D A, Oosterhuis D M, Gealy D R, Moss S R, Burgos N R, Miller M R and Palhano M 2016 Cultivars to face climate change effects on crops and weeds: a review Agron. Sustain. Dev. 36 12
Lesk C, Rowhani P and Ramankutty N 2016 Influence of extreme weather disasters on global crop production Nature 529 84–7
Müller C, Elliott J, Chryssanthacopoulos J, Deryng D, Folberth C, Pugh T A M and Schmid E 2015 Implications of climate mitigation for future agricultural production ERL 10 125004
Myers S S, Zanobetti A, Kloog I and Huybers P 2014 Increasing CO2 threatens human nutrition Nature
Potts S G, Imperatriz-Fonseca V, Ngo H T, Aizen M A, Biesmeijer J C, Breeze T D, Dicks L V, Garibaldi L A, Hill R, Settele J and Vanbergen A J 2016 Safeguarding pollinators and their values to human well-being Nature
Ray D K, Gerber J S, MacDonald G K and West P C 2015 Climate variation explains a third of global crop yield variability Nature Communications 6 5989
Rippke U, Ramirez-Villegas J, Jarvis A, Vermeulen S J, Parker L, Mer F, Diekkrüger B, Challinor A J and Howden M 2016 Timescales of transformational climate change adaptation in sub-Saharan African agriculture Nature Climate Change 6 605–9
Schleussner C-F, Lissner T K, Fischer E M, Wohland J, Perrette M, Golly A, Rogelj J, Childers K, Schewe J, Frieler K, Mengel M, Hare W L and Schaeffer M 2016 Differential climate impacts for policy-relevant limits to global warming: the case of 1.5°C and 2°C Earth System Dynamics 7 327–51
Serdeczny O, Adams S, Baarsch F, Coumou D, Robinson A, Hare W L, Schaeffer M, Perrette M and Reinhardt J 2016 Climate change impacts in Sub-Saharan Africa: from physical changes to their social repercussions Reg Environ Change 1–16
Settele J, Bishop J and Potts S G 2016 Climate change impacts on pollination Nature Plants 2 16092
Trnka M, Olesen J E, Kersebaum K C, Rötter R P, Brázdil R, Eitzinger J, Jansen S, Skjelvåg A O, Peltonen-Sainio P, Hlavinka P, Balek J, Eckersten H, Gobin A, Vucetic V, Marta A D, Orlandini S, Alexandrov V, Semeradova D, Šteěpánek P, Svobodová E and Rajdl K 2016 Changing regional weathercrop yield relationships across Europe between 1901 and 2012 CRes 70 195–214